3: Physical chemistry of crystal growth
Much of this section is specific to crystals grown from solution because that is how most samples seen in the UKy X-ray lab. were grown. After all, most synthetic chemistry is done in solution. In this regard, before we have crystals, we need material in solution, hopefully a fairly pure solution. There are three main steps:
• Nucleation
• Growth
• End of growth
To get crystals, they first have to nucleate. This is the initial formation of ordered aggregates that are large enough to continue growing rather than simply re-dissolve. Once stable crystal nuclei have formed, they grow by deposition of material from solution onto the surfaces of the nascent crystallites. At some point the crystals stop growing, which could be due to depletion of material in solution, or to poisoning of crystal surfaces by impurities, defects etc.
Crystal growth is governed by thermodynamics and kinetics. Given a compound in solution, the solution must first become saturated, at which point the system is at equilibrium. To initiate crystal growth, it must be perturbed in some way, so as to achieve supersaturation. In effect, the chemical potential of material in solution must be raised until it is higher than it would be in the solid phase. This establishes a driving force for precipitation, which proceeds until equilibrium is re-established. To gain insight into how and why crystals grow and also into how we might control the process, we need to define some terms.
Saturated: μicrys = μisol = μio
+ RT lnγi ci
This means that in a saturated solution, the chemical potential, μ, of species i is the same in solution as in the crystalline state. Here, R is the familiar universal gas constant, which is ubiquitous in physical chemistry, and T is temperature. The quantity γi is the activity coefficient of species i and ci is its concentration. In other words, it states that solution and solid phases are at equilibrium.
Supersaturated: μisol > μicrys
In a supersaturated solution, the chemical potential of species i is higher in the solution phase than in the solid phase. There will thus be a driving force to attain equilibrium, which causes deposition of solid until equilibrium is re-established.
Homogeneous vs heterogeneous
Nucleation may be homogeneous, occurring directly from solution, or heterogeneous, occurring on a substrate, such as a surface, interface, a speck of dirt, a small 'seed' crystal, or other nucleation site.
Supersaturation is a requirement for nucleation, but it does not just happen. It is possible to get supersaturated solutions that do not spontaneously crystallize. This is because there is an energy barrier, ΔGn, to nucleation:
ΔGn = [-kT(4πr3)/Vlnβ] + 4πr2γ
k = Boltzmann's constant
β = degree of supersaturation
γ = interfacial free energy between nucleus and solution
r = effective radius of crystal nucleus
V = molecular volume
The expression has two parts: a volume term (∝r3) and a surface area term (∝r2). The volume term is negative, and represents the advantage that results from the decrease in free energy of the system. The positive surface area term corresponds to the energy needed for deposition onto the surface. If we hold all terms other than r constant, we can visualize the energy barrier:
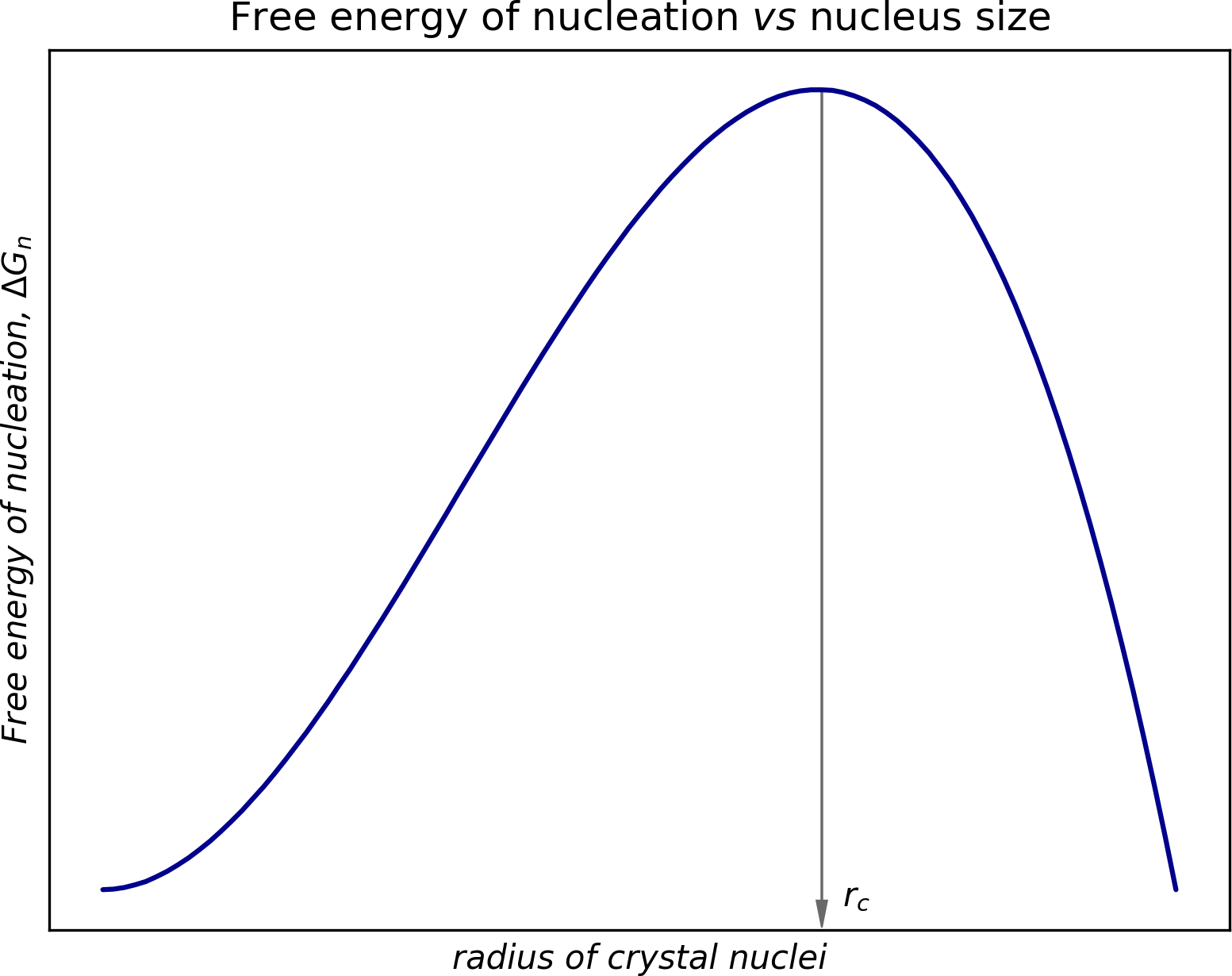
At first, ΔGn increases with r until it reaches a threshold, rc. After this, ΔGn decreases as r gets larger. Thus, crystal nuclei tend to redissolve if they are smaller than some critical size but will grow if they happen to get large enough. Supersaturation results in a higher mean free energy for the system. Fluctuations about this mean free energy greater than ΔGn might result in nucleation.
Consider a simplified phase diagram for crystallization from solution:
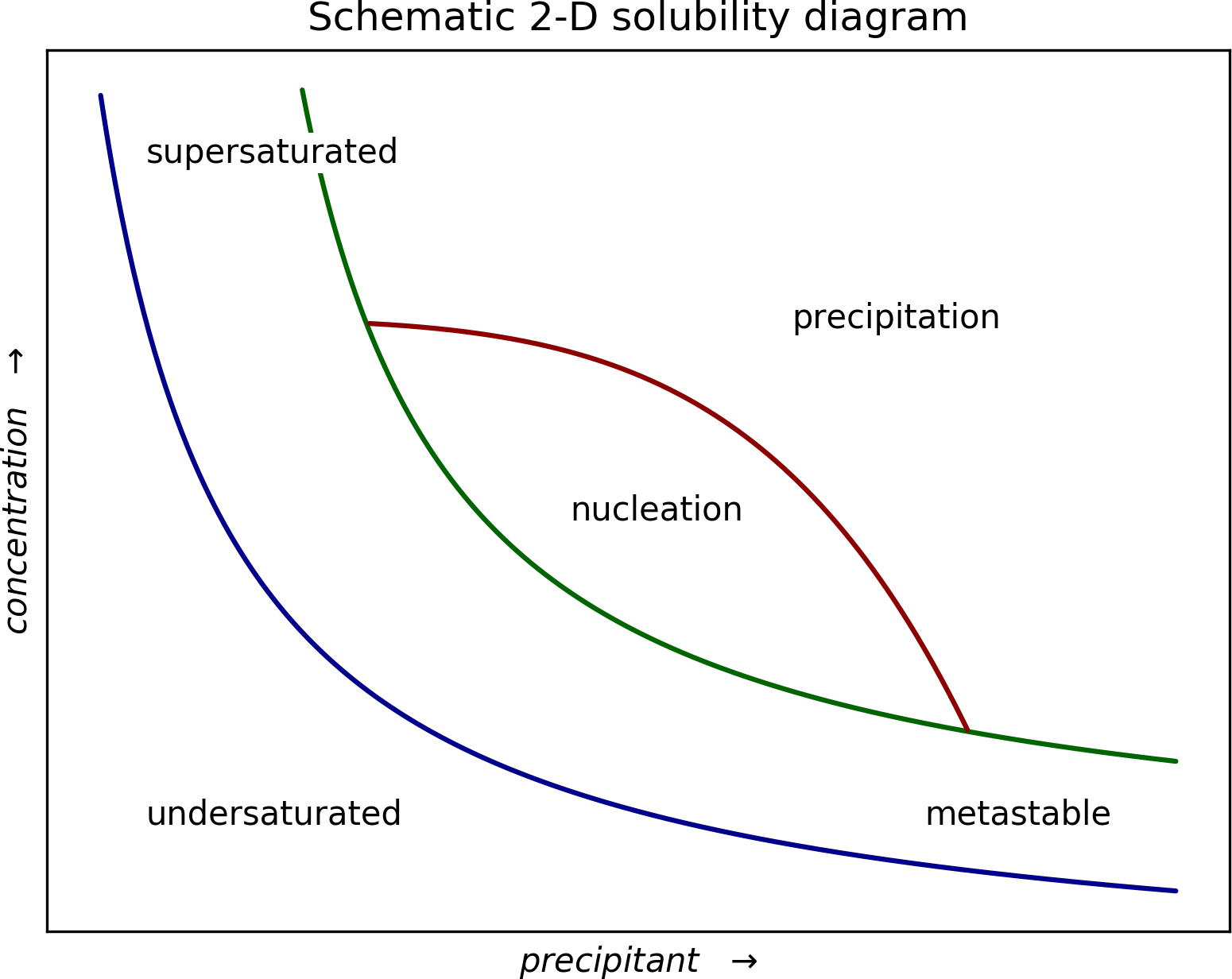
The slopes of the curved dividing lines will certainly change from system to system. Similarly, the axis labels might not be appropriate for different crystal growth regimes, but the concepts are valid for many systems. To nucleate the system must pass into the nucleation zone. Crystal growth can occur in the metastable region between the blue and green curved lines and equilibrium is re-established on passing back to the blue curve, at which point crystal growth stops. If the system drops below the blue curve, the crystals tend to re-dissolve.
A few additional points:
We probably don’t want to zoom far into the nucleation zone, as that would likely lead to a
shower of tiny crystals too small to use. We don’t want to have too high a concentration to
begin with, as then we'd run the risk of crystals growing too big or growing into each other
etc.
For crystal growth from the melt, the crystallization phase schematic would be a little different, as the parameters unique to solutions (e.g., degrees of saturation etc.) do not apply. Crystals form and grow at and below the melting point. Nevertheless, the general concepts are similar: if the cooling rate is slow enough, a few stable nuclei might form and grow as single crystals. As the cooling rate increases, nucleation might occur too rapidly, resulting in a shower of crystals that adhere to give a polycrystalline aggregate. For much too rapid cooling, the result might even be a non-crystalline glass.
In addition to thermodynamics there are also kinetic factors. Nucleation rates, for example, can be defined in terms of 'sticking probabilities'. If we define the nucleation rate as:
Jn = Bsexp(-ΔGn/kT)
Where Bs is a function of solubility and other unpredictable kinetic parameters related to diffusion coefficients etc., then we can make a few general predictions, as follows:
• High solubility leads to faster nucleation because there are more collisions and therefore more opportunity for species to stick.
• High supersaturation leads to reduced ΔGn because the supersaturation term β is in the denominator of the volume term used to define ΔGn.
• Heterogeneity in the form of a second phase, such as a surface or other interface, dust, scratched glass, etc. reduces the interfacial free energy γ in the expression for ΔGn. This explains why heterogeneous nucleation is more common than homogeneous.
Under the right conditions, once stable crystal nuclei have formed, the incipient crystals will grow. The particular mechanisms by which this occurs depend on characteristics of the system, as we'll see in the next section.
2: Crystal size, shape, and quality
3: Physical chemistry of crystal growth
4: Crystal growth mechanisms
Return to the main Tutorials page or to the main X-Ray Lab page